The Antiplasmodial Activities of Bufonidae (Toad) Venom Crude Extract on Plasmodium Berghei in Swiss Albino Mice by Ayim JO* in Open Access Journal of Biogeneric Science and Research
Abstract
Malaria remains one of the most prevalent and ravaging infection across Africa especially in Nigeria. Malaria parasite success or proliferation in its host have been linked to the ability of the parasite to show resistance to diverse anti-malarial drugs, thereby leading to the utilization of natural products from animals and plants as a potential strategy for identifying novel antimalarial drugs. Thus, this study aimed at screening toad venom for its antiplasmodial potency in swiss albino mice. Toads were collected from July to August 2019. The acute oral toxicity and biochemical characterization of the toad venom was determined. The experimental mice was administered varying curative doses (130, 173 and 217 mg/kg) of the toad venom crude extract and standard drug once daily for 3 days. In-vivo antiplasmodial activity was evaluated by a curative test, after infecting the mice model with Plasmodium berghei. From the results obtained, parasitemia was highest in the control group while the group treated with Artemether-lumerfantrin (ACT) had the least parasitemia. Thus, the mean parasitemia in relation to the different treatments varied significantly (P < 0.05). Nevertheless, the mean parasitemia in albino mice in relation to the highest dosage of toad venom versus ACT treatments showed no significant difference (P > 0.05). The mean change in haemoglobin (Hb) and as well as pack cell volume (PCV) levels in albino mice after four days in relation to treatments with toad venom and ACT showed a significant difference (P < 0.05) whereasthe red blood cell (RBC) level did not vary (P > 0.05). This study shows that the toad venom reduced the parasitemia in the group that was treated with 217 mg/kg toad venom which is thus comparable to the group treated with 8mg/kg standard drug (ACT). Sequel to the potency demonstrated by toad venom against plasmodial activities, it is crucial to generate a reliable and economic bufo-genic therapeutic product, while being conscious of the protection of these species. The study recommends an affirmatory research in relation to the isolation and characterization of the active anti-plasmodial agents responsible for the curative activity observed and as well as consider species-specific active compounds.
Keywords: Bufonidae; acute oral toxicity; biochemical characterization of toad venom; antiplasmodial potency of toad venom; Plasmodium berghi; Swiss albino mice; haematological parameters
Introduction
Malaria disease is characterize by a flu-like illness, accompanied by fever and chills; that may be fatal, if not treated [1]. It is caused by a protozoan parasite of the genus Plasmodium [2]. Malaria disease contributes greatly to the world disease burden and accounts for many death cases recorded in hospitals. According to a recent World malaria report released in February 2020, there were 229 million cases of malaria in 2019, up from 228 million cases in 2018 and 219 million cases in 2017 respectively [3,4]. The estimated number of malaria deaths stood at 435 000 in 2017, a similar number to the previous year. Nigeria among other African countries recorded more death cases due to malaria with about 25% malaria occurrence [1]. This is apparently an indication that malaria is highly endemic in Nigeria. This presents a severe economic and health burden to the country with about 97% of the population at risk of infection [5].
Four species of Plasmodium are recognized to cause malaria in man, this includes: Plasmodium falciparum, P. vivax, P. malariae, and P. ovale, transmitted by over 70 Anopheles species. Among the malaria parasites, P. falciparum is the most severe and blamable for 90% of all malaria deaths [6]. P. berghei have been described in African murine malaria with others being Plasmodium chabaudi, Plasmodium vinckei and Plasmodium yoelii. Due to P. berghei ability to infect rodents and the relative ease of genetic engineering, it is thus used as a well-known model organism for the investigation of malaria in man [7]. This makes this species efficient in research programs for development and screening of anti-malarial drugs and malaria vaccines that are effective [8].
Plasmodium’s life cycle is intricate, involving many developmental stages and sites, both in the vector and the animal host [9]. Although its intricate infectious cycle presents several sites for the development of specific drugs, finding a universal drug against malaria has been a perplexing task [10]. The main problem with the presently available anti-malarial drugs is the constant resistance developed by some parasites as opined by [4, 11-14]. The mode of action of the drugs used at the present time for the treatment of some parasitic infections are yet not very known, aiding mutations and initiating drug resistance by the parasites [15-18]. Artemisinin resistance has been described by White [12] and Dondorp et al. [19] in malaria endemic areas, threatening malaria control, treatment, and eradication of global efforts. As it is arising with anti-bacterial drugs, the abuse and the mono-therapeutic use of anti-parasitic drugs is also leading to resistance, increasing preoccupation about a growing arrival of new cases and inefficacy on the treatment of recurrent cases [12, 19-21]. The utilization of natural products has delivered a potential strategy for identifying novel antimalarial drugs. The utilization of animals, fungi, bacteria and plants are important springs of biologically active substances with structural variety and novel mechanisms of action, which can possibly provide patentable products [22-25].
The skin secretions and venom of amphibians are rich sources of bioactive compounds, such as peptides, bufadienolides, alkaloids, proteins and biogenic amines, which have contributed meaningfully to human health and most pharmaceutical researches [26,27]. Yang et al. [27] maintained that the toad toxins from the parotoid or skin glands have significant therapeutic value for a good number of diseases. Against this backdrop, this study screened toad venoms for its antiplasmodial potency in Swiss albino mice infected with P. berghei.
Material and Methods
Toads Collection
Toads were collected from July to August 2019 between the hours of 0700 and 1000 from rice fields located on Latitude 8º 35´0.09ʺ E and Longitude 8º 28´18.45ʺ N beside the fence of the Take-Off Site of Federal University of Lafia, Nasarawa State, Nigeria. They were collected into a ventilated container and conveyed to the Department of Zoology Laboratory in the Permanent Site of Federal University of Lafia for extraction.
Extraction Of Crude Venom Extract From Toad
The process of extraction of toad venom was according to Gao et al. [28]. The toad was massaged and then the parotoid macro-glandules was pressed out. The toad was then released to its natural environment. The collected secretion was lyophilized and stored in a freezer at the Federal University of Lafia.
Biochemical Characterization
Determination of the quantitative and qualitative chemical components of the venom was achieved by Mass Spectrometry using a Mass spectrophotometer at the Spectral Laboratory and Services, Tudun wada Kaduna South, Kaduna State, Nigeria. The Shimadzu Fourier transform Infrared Spectrophotometer- FTIR 8400 S was used for the determination of the functional groups present in the venom. Gas Chromatography Analysis of toad venom was done using gas chromatography (Perkin-Elmer 8500). The Scanning Electron Microscope energy dispersive X-ray spectroscopy (SEM-EDS) Phenom Prox, manufactured by phenom World Eindhoven (Netherlands) was used to carry out the morphology analysis (that is; analysis of the chemical elements present in the toad venom).
Experimental Animals
Twenty-five laboratory albino mice, weighing between 14g and 37g were purchased from National Veterinary Research Institute Vom, Plateau State. All animals were fed with formulated feeds and water was administered ad libitum. The animals were allowed to acclimatize for a period of 7 days prior to their randomization into the various experimental groups.
Coding And Weighing Of Animals
The mice were marked with a permanent ink markers on different anatomical parts of the body of each animal. These include, the head (HD), back (BK), tail (TL), right leg (RL), left leg (LL), right side (RS), left side (LS), right arm (RA), left arm (LA), back strip (BKST), head and back (HDBK), head and tail (HDTL), head and right leg (HDRL), head and left leg (HDLL), head and right side (HDRS), head and left side (HDLS), head and right arm (HDRA), head and left arm (HDLA), head and back strip (HDBKST), back and tail (BKTL), back and right leg (BKRL), back left leg (BKLL), back and right side (BKRS), back and left side (BKLS), back and right arm (BKRA). The weight of each animal was measured using the electronic compact scale.
Toxicity Study
The median oral lethal dose of the toad venom was determined in mice according to Lorke [29]. The toxicity test was carried out in two phases at the in-vivo laboratory of National Institute for Pharmaceutical Research and Development (NIPRID), Federal Capital Territory, Abuja, Nigeria.
Phase 1: Nine Swiss albino mice were divided into three groups with each having three individuals. The groups were administered varying doses of 10, 100 and 1000 mg/kg respectively of the toad venom and then placed under observation for 24hours in order to monitor their behaviour as well as mortality.
Phase 2: Another set of three animals, distributed into three groups of one animal each were administered varying doses of 250, 500 and 750 mg/kg respectively of the toad venom and observed for 24hours in relation to behaviour and mortality.
Then the LD50 was calculated by the formula by Lorke [29]:
LD50=√((D_0 x D_(100 )))
Where
D0 = Highest dose that gave no mortality,
D100 = Lowest dose that produced mortality.
Grouping, Infection And Treatment Of Infected Swiss Albino Mice
Twenty-five mice were marked and infected with Plasmodium berghei obtained from NIPRID and randomly divided into five groups of five mice per group. Parasites were maintained through serial blood passage in mice wherein the mice previously infected with Plasmodium berghei and with a high parasitemia level served as the donor. Blood samples was taken from the donor and diluted with phosphate-buffered saline such that 0.2 ml injected intraperitoneally into the experimental animals contained 1 × 107 infected erythrocytes. The blood of the mice were screened for infection before inoculation with parasite.
The curative method described by Ryley and Peters [30] was used. Treatment commenced following confirmation of parasitemia in the mice. Groups 1, 2 and 3 were treated with 217 mg/kg, 173 mg/kg and 130 mg/kg respectively of crude toad venom extract whereas group 4 mice were treated with 8 mg/kg of Artemisinin Combination Therapy (Arthemeter-lumerfantrin) tablet which was crushed and diluted in distilled water before administration, while those in group 5 were infected and received no treatment (control group). Administration of treatment was done orally once daily by gavage using a mouse feeding tube for four days for the standard drug used. The parasitemia of experimental mice was establish before every treatment was administered.
Determination of Parasitemia
Blood samples were collected by bleeding the mice through the tail vein. Thin blood smears were made on clean glass microscope slides. The films were dried in air and then fixed in methanol and stained with 10% Giemsa solution [31]. The stained film was then observed under the binocular compound microscope and viewed for parasitemia. The percentage parasitemia were determined by counting the number of parasitized red blood cells (pRBC) for at least six different fields.
Percentage Parasitemia = (Parasitized RBCs)/(Total RBCs) x 100
Hematological Parameters
Using the methods described by Cheesbrough [32], the Packed Cell Volume (PCV), haemoglobin (Hb) and erythrocyte (RBC) counts were determined. These parameters were determined for each mouse before infection and after treatment. Blood samples were collected from the tail of each mouse with a heparinized capillary tube with one end sealed with plasticine.
Data Analysis
Data obtained were analyzed using R Console software (Version 3.2.2). Shapiro-wilk normality test was carried out and the data was observed to be normally distributed. One-way analysis of variance (ANOVA) was used to compare the mean parasitemia in albino mice in relation to toad venom and ACT treatments. Also, mean change in body weight and as well in haematological parameters were compared using one-way ANOVA. ANOVA test was followed by a post-hoc Tukey’s Honest Significant Difference (Tukey HSD) test where there was significant difference between the means of the treatments. The level of significance was set at P < 0.05.
Table 1: Analysis of the chemical components of toad venom crude extract using the GC-MS.

Table 2: Protein and non-protein functional groups present in toad venom crude extract at different peaks using the FTIR.
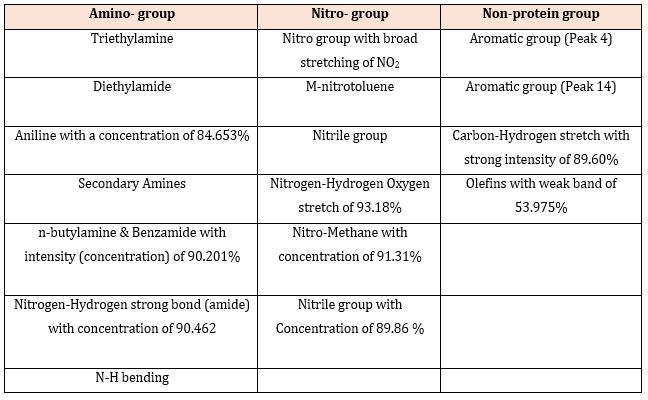
Table 3: Analysis of chemical elements present in the toad venom crude extract using the SEM-EDS.


Figure 1: Mass spectroscopy of toad venom crude extract showing the abundance of the chemical compounds at different peaks.

Figure 2: The functional groups present in the toad venom crude extract at different peaks.

Figure 3: Mean parasitemia in Swiss albino mice in relation to treatments with toad venom and ACT.
Results
Determination of the Biochemical Compounds in the Toad Venom Crude Extract
The results of the biochemical analysis of the toad venom crude extract showed that 9,12-Octadecadienoic acid (Z,Z) compound was the most dominant at peak 3 as shown in Figure 1 which had a rate of 38.615 at a proportion of 49.76% (Table 1) followed by n-Hexadecanoic acid (29.04%) then Octadecanoic acid (7.03%), Squalene (5.48%), 1-Hexadecyne (3.87%), Butyl 9,12-octadecadienoate (2.95%) while Hexadecanedioic acid was the least compound (1.87%). (Figure 2) showed that the toad venom crude extract contains 18 active functional groups in which Nitrogen-Hydrogen Oxygen stretch of 93.18% was the most active functional group. The toad venom crude extract was predominantly characterized by the presence of the amino group made up of 8 functional groups, followed by the nitro-group having 6 functional groups while the non-protein group was made up of only 4 functional group as shown in Table 2.
The SEM-EDS analysis revealed that the toad venom crude extract contains 11 chemical elements, out of which carbon had the highest atomic concentration and weight of 61.76 mol/dm3 and 41.04 g/mol respectively, followed by oxygen (25.04 mol/dm3 and 22.29 g/mol) while iodine and aluminium had the least atomic concentration and weight of 0.24 mol/dm3 and 0.90g/mol respectively (Table 3).
Determination Of The Oral Toxicity (Ld50) Of The Toad Venom Crude Extract
The oral toxicity experiment conducted using the Lorke’s method indicated that the toad crude venom extract caused mortality at a dose of 1000 mg/kg after one hour. Hyperactivity, convulsion and constant stooling were observed prior to the death of the animals. However, on the administration of 750 mg/kg, hyperactivity, diarrhea and calmness were observe within one hour, but there was no mortality recorded. The oral toxicity of the venom established was 866 mg/kg.
Mean Parasitemia In Swiss Albino Mice Across Treatments With Toad Venom And Act
The overall parasitemia was highest in the control group and least in the group treated with ACT. Therefore, the mean parasitemia in albino mice in relation to treatments showed a very high significant difference (F120 = 10.34, Adjusted R2 = 0.2315, P < 0.0001, Figure 3). A closer look at the parasitemia in albino mice between those treated with the highest dosage of toad venom and ACT treatments showed no significant difference (P > 0.05) as shown in Table 4.
Change In Body Weight Of Swiss Albino Mice After Four Days Of Treatment
After the treatments, the highest weight gain was observed in treatment with 8 mg/kg ACT (Group 5) and the least weight in the control group (infected but not treated). Thus, the mean change in body weight of albino mice after four days of parasitemia treatment in relation to treatments with toad venom and ACT showed a very high significant difference (F20 = 6.694, Adjusted R2 = 0.4869, P = 0.001374), (Figure 4, Table 5).
Mean change in haematological conditions of albino mice after four days of treatment:
Hemoglobin (Hb)
The mean change in Hb level in albino mice after four days in relation to treatments with toad venom and ACT showed a significant difference (F10 = 4.863, Adjusted R2 = 0.5246, P = 0.01942), (Table 6, Figure 5).
Mean Change In Red Blood Cell
The mean change in RBC level in albino mice after four days of parasitemia treatment in relation to treatments with toad venom and ACT showed no significant difference (F10 = 1.929, Adjusted R2 = 0.2098, P = 0.1821, Table 6, Figure 6).
Mean Change In The Pcv Level
The mean change in PCV level in albino mice after four days of parasitemia treatment in relation to treatments with toad venom and ACT showed a very high significant difference (F10 = 6.014, Adjusted R2 = 0.5889, P = 0.009893, Table 6, Figure 7).
Table 4: Turkey multiple comparisons of means of parasitemia 95% family-wise confidence level.

Table 5: Tukey multiple comparisons of means for change in body weight between treatments 95% family-wise confidence level.

Table 6: Effects of toad venom extract on the haematological parameters of Albino mice, Values were presented as Mean ± SE; n= 5 for each group.

Table 6: Hierarchical Regression Results.

Figure 4: Mean change in body weight of albino mice in relation to treatments with toad venom and ACT after four days.

Figure 5: Mean change in hemoglobin level in albino mice in relation to treatments with toad venom and ACT after four days.

Figure 6: Mean change in RBC level in albino mice in relation to treatments with toad venom and ACT after four days.

Figure 7: Mean change in PCV level in albino mice in relation to treatments with toad venom and ACT after four days.
Discussion
The biochemical analysis revealed the presence of 9,12-Octadecadienoic acid (Z,Z-) and n-Hexadecanoic acid in the toad venom which is consistent with the findings of Abubakar and Majinda [33] who recorded similar compounds from a GC-MS analysis and the preliminary antimicrobial activity of Albizia adianthifolia and Pterocarpus angolensis. Among other compounds isolated, 9,12-Octadecadienoic acid (Z,Z-) and n-Hexadecanoic acid showed the best antimicrobial activity against E. coli. The presence of squalene, in this study is symbolic of the antitumor potency [34] of the venom and thus, the assurance of little or no cytotoxic activity in vivo. Opinions from Lozano-Grande et al. [35] suggests that squalene is a chemotherapeutic and chemo-preventive agent which inhibits tumor growth and stimulate the immune system in the treatment of diseases.
Amino compounds like Triethylamine, Diethylamide and Aniline that were observed in this study agrees with the findings of Krieg et al. [36] who characterized arymethylamino steroids as a class of compounds with remarkable activity against P. berghei in mice and P. falciparum and Schistosomes (in vitro). It may be inferred that the parasite inhibition observed in this study may be as a result of the presence of these active proteins.
The presence of Carbon, Nitrogen and Oxygen is indicative that these elements make up a significant portion of the nucleic acid, amino acid, sugars and fatty acid molecules found in the toad venom [37].
The experimentation of the oral toxicity (LD50) of the toad venom crude extract indicates that the venom becomes lethal to the laboratory albino mice at a dose of 866 mg/kg. Clinical signs of toxicity observed includes: hype-reactivity, diarrhea, sedation, convulsion, constant stooling and death. The presence of lead and Chromium in the toad venom recorded in this study could have accounted for the mortality observed in the mice [38]. This agrees with Arena and Drew [39] who opined that the oral toxicity of toad venom is 0.36mg/kg and the common adult clinical dosage is 3-5mg/day, thus it becomes lethal above a dose of 135 mg/day. Although, the administration of 130 mg/kg in this study as a curative dose yielded no mortality. Similarly, this is consistent with that of Gadelha et al. [40] who observed no clinical signs of toxicity or even death on the administration of 25 mg/kg dose.
Parasitemia in Swiss albino mice decreased within the four days of treatment (Figure 3). This possibly suggests that the toad venom would be a good source of antiplasmodial drug because the parasitemia in the albino mice was almost two times less in comparison to those in control group. This concurs with the finding of Banfi et al. [41] who experimented the in-vitro antimalarial activities of compounds isolated from Rhinella marina venom (cane toad) and observed it was effective and efficient against P. falciparum. Banfi and colleagues isolated Marinobufotoxin, Dehydrobufotenin, Marinobufagin and bufalin, out of which the Dehydrobufotenin revealed to be most active against malaria and showed the absence of cytotoxicity in-vitro. This finding is also in tandem with that of Gao et al. [28] who revealed that peptides isolated from scorpion venoms are active against Plasmodium species.
The low parasitemia observed in the group treated with ACT (Artemether-lumerfantrin) clearly showed that it is effective in the control of malaria parasites in animals. This is in agreement with the findings of Ihekwereme et al. [42] who reported parasite inhibition in P. berghei infected mice treated with the standard drug (artemether-lumefantrin). Similarly, Nyandwaro et al. [43] demonstrated the efficacy of the standard drugs in the treatment of malaria in Swiss albino.
The lack of variation in parasitemia in albino mice in relation to the highest dosage of toad venom and ACT treatments probably shows that the toad venom can serve as an alternative to the standard drug (ACT) when administered at a higher dosage since ACT was formulated primarily against Plasmodium falciparum in man. Correspondingly, it can be argued that chloroquine has been used for many decades against malaria in man and it is also active against murine malaria. It is therefore worthy of note that the P. berghei is sensitive to chloroquine [44-46]. The effective inhibition of the toad venom against P. beghei, in-vivo is in tandem with a recent finding by Banfi et al. [47] in Brazil who demonstrated an in-vitro inhibition of Plasmidium falciparum using toad venom. This is a further reflection of the isolation of peptides from animal venoms against protozoan infections, as the peptides scorpine isolated from scorpion venom was responsible for the mortality of P. berghei gametocytes at 15µm and 100% reduction of P. falciparum at 5µm [48].
Hematological parameters like hemoglobin (HBG), red blood cell (RBC) count and packed cell volume (PCV) are indicative of anemia in Plasmodium parasitized individuals [49]. Consequently, this research considered changes in the hematological conditions of Swiss albino mice after four days of malaria infection and associated treatments. The observed changes in hemoglobin level in albino mice after four days of parasitemia treatment in relation to treatments suggests that the toad venoms contains some anti-anemic components. This is similar with the findings Banfi et al. [41] who recorded a drastic reduction in cytotoxity on the use of compounds isolated from the cane toads. The highest hemoglobin values were observed in the group 4 (217mg/kg venom) and the least was observed in the control group this may be as a result of the phagocytic action of the parasite on haemaglobin, consequently digesting it into haem and globulin for its consumption. This concurs with the findings of Cyril-Olutayo et al. [50] and Mokennem [51] who recorded an improved haematological parameters on the use of extracts from Anogeissus leiocapus and Croton species respectively, in-vivo.
The lack of variation in the mean change in RBC levels in the mice after four days of parasitemia treatment in relation to the treatment with toad venom and ACT possibly suggests that parasite targets the RBCs despite treatment. On the average, the untreated group had the least number of RBC value as a result of damage of the red cells caused by the erythrocytic stages of the malaria parasites [52,53].
The variation in the mean change in PCV level in Swiss albino mice after four days of parasitemia treatment in relation to treatment with toad venom and ACT may be as a result of the parasite inhibition pace and varying response in the experimental mice. Although the infected and untreated group had the least PCV value probably due to the destruction of red blood cells which in turn affected the percentage of the packed cells. This further shows that the reduced percentage of PCV is an indication of anaemia in malaria infected individuals. However, improved PCV values were observed in both the treatments with toad venom and ACT [54].
Conclusion
Malaria parasite success or proliferation in its host have been linked to the ability of the parasite to show resistance to host immune defense and the diverse anti-malarial drugs. However, this study has shown that pharmacological foundation of toad venom have some antiplasmodial effect against the non-human form of the parasite and could therefore be further investigated for use in the development of prospective drug for the treatment of malaria. From this findings, it is observed that the effectiveness of toad venoms at a very high dose (as was used in this study) was comparable to that of ACT. Hence, it supports the use of toad venoms in folk medicine as it is practiced by the Chinese.
Regardless of the therapeutic contributions of the bufo-genic products in medicine and Public health, there is still a limited research and manufacture of toad-derived drugs. This study therefore recommends the exploration of these potent chemicals in the treatment of parasitic ailments following appropriate quantification and modification by pharmaceutical companies. Also, an affirmatory research, both in-vivo and in-vitro proceeded by the isolation and characterization of the active anti-plasmodial agents responsible for the curative activity observed and considering species-specific active compounds should be carried out.
More information regarding this Article visit: OAJBGSR
No comments:
Post a Comment